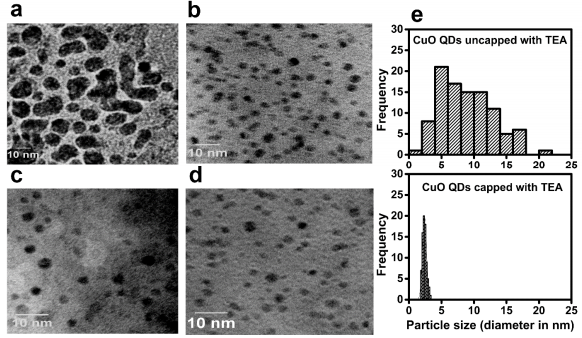
The Applied Nanostructures Engineering and Nanochemistry (ANEN) Research Group
Can we use mercury containing contaminated water to fuel the planet while cleaning it up?
Hydrogen is truly the best fuel; it can offer almost 141 kJ for each gram. Diesel and petrol/gasoline merely have 1/3rd of this calorific value. However widespread use is fraught with many difficulties. The challenges currently with usage of hydrogen are to do with safety concerns in gas storage and transportation, and low rate of production leading to non-viability of technologies at the point-of-use. Another global concern of immediate relevance involves heavy-metal (say mercury i.e. Hg) ion pollution. Viable processes which can simultaneously remove and result in beneficiation of the contaminants are almost never reported. It is in the above context that we have developed a single-step, in situ co-reduction approach which has the dual advantage of (i) Hg contaminant removal, and (ii) room temperature hydrogen production. The key component resulting in hydrogen generation is a nano-amalgam that is produced within the “dirty” water. The hydrogen production rate (720 mL/min for 0.5 g-Al salt) is far superior to what would be expected from the use of pure hydrides, and/or using bulk amalgams at room temperature. The method we have developed is simple, chimie douce (i.e soft chemical), hence potentially affordable, and capable of providing a means of beneficiating Hg contaminated water present in effluents from certain industries (for example, industries which uses chlor-alkali process). The in situ co-reduction approach helps in bypassing the usual rate limiting steps. Given the potential that exists in scale down and up, this approach offers a method to address the long standing challenge of point-of-use hydrogen availability. Think about it – using dirty water as a source of fuel need not be in science fiction anymore!
For more information please read our patents and paper referred here.
Ref: Abdul Malek, Edamana Prasad, and Tiju Thomas, “Chimie douce hydrogen production from Hg contaminated water, with desirable throughput, and simultaneous Hg-removal”, International Journal of Hydrogen Energy (2017; https://doi.org/10.1016/j.ijhydene.2017.05.082) “Hydrogen generation from waste water via galvanic corrosion of in-situ formed aluminum amalgam” (2016) (Indian Patent application no. 201641027502) Copper oxide quantum dots - one size fits all here (when using our size focussing process)! They are stable and plausibly useful too.
Ultra-small stable quantum dots (essentially extremely tiny particles that confine even the free electrons in them rather well) are very interesting for a wide range of applications. However using them for applications requires them to be of essentially the same size which is rather hard. However Niya Mary from our group discovered a size focusing mechanism in ceramic systems (ZnO to be precise). Bhusankar from the group has extended this result to copper oxide as well. However unlike ZnO; down at this size regime Cu seems to flip-flop between two different oxidation states. Fascinatingly the usual formula that works for quantum dots does not work for our materials; we suspect this has to do with the nature of Cu in these systems (i.e it being of multiple oxidation states; namely a mix of +1 and +2).
Ongoing efforts indicate that the size-focusing approaches we have developed are primarily dependent on specific interactions between the Cu atom and the surface-active agent (called hard-hard-acid-base interactions) used in this process; the solvent we use matters too.
In fact there are ongoing theoretical and computation efforts to develop predictive models for this process so that the experiments may be intelligently designed.
Ref: Bhusankar Talluri, Edamana Prasad, and Tiju Thomas, “Ultra-small (r<2 nm), stable (>1 year), mixed valence copper oxide quantum dots with anomalous band gap”, arXiv:1706.01261 (2017)
“Sniffing” hydrocarbons using low dimensional material based devices?
This is how the device is made (Thanks to Aneesh from IITB and Dr. Maity from Tezpur University). The idea here was to make a device that can sense (i.e sniff!) methane; which is a hydrocarbon that is rather hard to detect. However detecting it would be nice since beyond a certain concentration it can be explosive in air.
This is how the low dimensional material structure relevant to this “sniffer” is made. The device material is essentially just slender graphene oxide (essentially just a carbon paper that is a few atomic layers thick with a few oxygen atoms here and there) along with zinc oxide tiny particles (nanoparticles). Appropriate heat treatment is very essential to get a good device material (in other words; they better be cooked well – like in case of food under or over cooking is problematic).
At the site of action – methane – an otherwise difficult to detect molecule is oxidized (essentially burnt up); the electronic signatures of which are helpful in sniffing out its presence. ZnO seems to aid and accelerate this oxidation process resulting in higher sensitivity of this device and quicker response.
Ref: Argha Sarkar, Santanu Maity, Aneesh M. Joseph, S Chakraborty, and Tiju Thomas, “Methane-Sensing Performance Enhancement in Graphene Oxide/Mg:ZnO Heterostructure Devices” Journal of Electronic Materials (2017); DOI: 10.1007/s11664-017-5619-1
Nitrogen can play a “good guy” when locked into a solid that taps sunlight In this string of publications we showed that nitrogen; that ever present gas that constitutes almost 78% of dry air in our atmosphere, is a friend when locked inside a solid. TiO2, a very well respected “light harvester” (fancily called photoactive material), does even better with nitrogen in it. For introducing nitrogen into the material we use a rather simple process wherein the material is merely “cooked” in an ammonia containing atmosphere. The cookware used is called “alumina boat” and the stove used is called a “tubular furnace”. Of course the cooking is often done at temperature that are somewhat hot (>400oC). We also show that making TiO2 rather spongy (i.e porous) is simple. We just use basic colloidal science which you perhaps already know; to achieve this. The basic idea is to use some “soaps” that come together while holding onto Ti containing units. When cooked; outcomes spongy TiO2 (called nanoporous TiO2 due to the tiny pores it contains). This porous material soaks up nitrogen rather efficiently and hence “doping” nitrogen into this form of TiO2 is a piece of cake (figuratively speaking of course). Materials scientists (i.e our breed) are rarely satisfied with a first order investigation of the above mentioned kind. So we went onto study various combinations of N doped TiO2 with (i) Ag (essentially painted on these materials), (ii) Fe as a co-dopant. In another set of experiments we went beyond TiO2 and showed that Cu2O can be a competitive light harvester as well; interestingly particles with sharp edged seemed to help; once again nitrogen was a friend here.
Ag coated TiO2 (doped with nitrogen) looks nifty and also does a good job with light harvesting and conversion. Interestingly use of Ag not just enhances light absorption but also helps with light conversion (likely due to small silver oxide/TiO2 junctions on these tiny surfaces!).The technique used for making these meso- and nano-porous materials is sufficiently generic and could be useful for several others materials too. Refs: Mingming Zou, Fengqiang Xiong, Ayyakannu Sundaram Ganeshraja, Xiaohua.Feng, Chuanxi.Wang, Tiju Thomas∗ and Minghui Yang, Visible light photocatalysts (Fe, N):TiO2 from ammonothermally processed, solvothermal self-assembly derived Fe-TiO2 mesoporous microspheres”, Materials Chemistry and Physics (2017; just accepted) 10.1016/j.matchemphys.2017.04.035 Zou Mingming, Honghong Liu, Lu Feng, Tiju Thomas, and Minghui Yang, “Enhanced visible light photocatalytic activity in N-doped edge-and corner-truncated octahedral Cu 2O”, Solid State Sciences 65, 22-28 (2017) Zou Mingming, Honghong Liu, Lu Feng, Fengqiang Xiong, Tiju Thomas, and Minghui Yang. “Effect of nitridation on visible light photocatalytic behavior of microporous (Ag, Ag2O) co-loaded TiO2”, Microporous and Mesoporous Materials 240 (2017): 137-144.
In separate investigations we found that oxynitride materials which are systems that contain specific atomic ratios of oxygen and nitrogen are rather finicky. They really care about the starting materials and what fraction of the tapped sunlight is available to us depends very much on how we begin the process. Some physical property studies of these materials showed that this was because the number of charge carriers in these oxynitrides vary dramatically based on the starting materials. In fact making these systems is currently an art; and we certainly enjoy this art form ☺
LaTiO2N formed using our methods are rather nanoporous; providing plenty of opportunities for use in catalytic and sensing applications. In this work we used this material for using light to split water (in a process called photoelectrochemical water splitting).
How one goes about making LaTiO2N determines the efficiency with which the light harvested is useful for splitting water.
We are currently looking at both the theoretical/computational and experimental aspects of this art. In particular we are developing computational approaches to see how these materials behave when heated up. In particular material interfaces, their evolution, and emergent electronic properties are currently being pursued by Kousika Anbalgam and Santosh who are graduate students in the group. Ref: Ref: Wan, Lipeng, Feng-Qiang Xiong, Yue Li, Tiju Thomas, Ruxin Che, and Minghui Yang. “Low Defect Density, High Surface Area LaNbON2 Prepared via Nitridation of La3NbO7” Materials Letters 188, 212–214 (2017). http://dx.doi.org/10.1016/j.matlet.2016.11.012 Xiong, Feng-Qiang, Lipeng Wan, Yue Li, Tiju Thomas, Francis Joseph DiSalvo, and Minghui Yang, “Crucial role of donor density in the performance of oxynitride perovskite LaTiO2N for photocatalytic water oxidation reaction”, ChemSusChem (2017) 10, no. 5 (2017): 930-937.
Fun bonus fact: Not surprisingly the magnetic ordering of these materials seems to be very sensitive to the amount of nitrogen in them. Briefly speaking the “when, where, how, and how-much” of magnetic and crystallographic transitions depend on presence of N in materials. We showed this using nickel chromates as the parent material. There is also evidence that these interesting dynamics in chromates has to do with the fact that the chromium atom gets into a “confused state” and exists between multiple oxidation states. Also any asymmetries in the crystal get quenches with increasing nitrogen; this correlates with substantial increase in covalent bonding in this system. Typically all magnetic transitions shift downwards in temperature with increase in nitrogen content. This correlates well with increases magnetic frustration in these doped chromates, with increase in nitrogen content.
Specific heat capacity which is a measure of how much heat is required to raise the temperature of the object by 1oC is a nice measure of transitions occurring in materials. This is in fact the way by which we explore crystallographic and magnetic transitions in these N doped chromates. Ref: Xin Liu, Nan Yin, Tiju Thomas, Minghui Yang, Junhu Wang and Quan Shi, “Effect of nitrogen substitution on the structural and magnetic ordering transitions of NiCr2O4”, RSC Advances 6, 112140-112147 (2016); DOI: 10.1039/C6RA22773B